Future of tech 5: Building a More Efficient World: The Power of Advanced Materials and Nanotechnology
- 3 days ago
- 19 min read
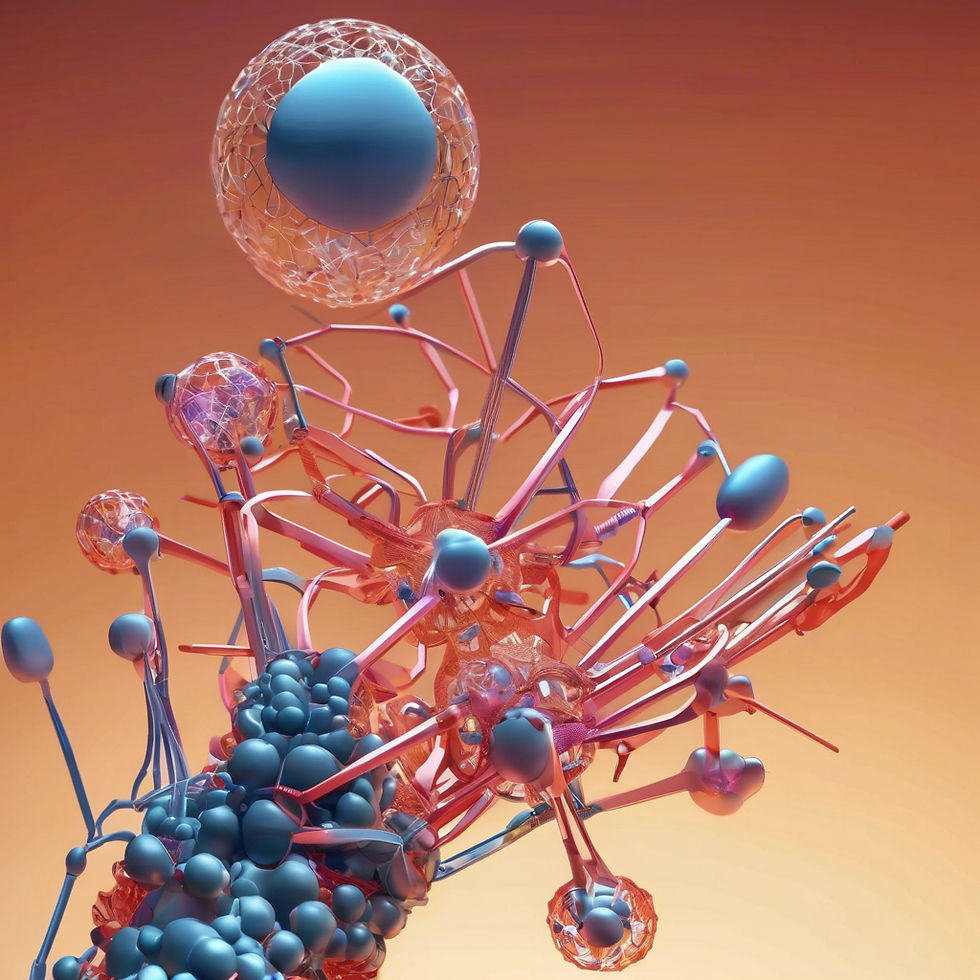
In an era defined by increasing global populations and finite resources, the pursuit of efficiency has become paramount across all sectors. From minimizing energy consumption to optimizing industrial processes and constructing resilient infrastructure, the need to do more with less is driving innovation at an unprecedented pace. At the forefront of this quest for efficiency are two closely related and rapidly evolving fields: advanced materials and nanotechnology. These cutting-edge disciplines are not only transforming the way we design and manufacture products but are also offering promising solutions to some of the world's most pressing challenges, paving the way for a more sustainable and efficient future. This detailed exploration will delve into the fundamental definitions and principles of advanced materials and nanotechnology, showcase their diverse applications in enhancing efficiency across various industries, analyze the potential environmental implications, and explore the exciting trends and breakthroughs that lie ahead.
Laying the Groundwork: Understanding the Building Blocks
Before examining their transformative applications, it is essential to establish a clear understanding of what constitutes advanced materials and nanotechnology.
Defining Advanced Materials: Properties and Illustrative Examples
The term "advanced materials" encompasses a broad range of materials that have been intentionally engineered to exhibit superior properties compared to their conventional counterparts. These materials are processed, synthesized, fabricated, and manufactured to achieve high-performance characteristics tailored for specific applications across structural, electronic, magnetic, or photonic domains, as well as for joining, welding, or bonding purposes. Various organizations and researchers offer definitions that highlight the key aspects of these innovative substances. For instance, advanced materials possess novel or enhanced mechanical, chemical, and physical properties, such as exceptional strength, durability, flexibility, and conductivity, often engineered at the nanoscale level. This nanoscale engineering is a recurring theme, underscoring the close relationship between advanced materials and nanotechnology.
While a universally agreed-upon definition remains elusive, the defining characteristic of advanced materials lies in their ability to outperform traditional materials in specific applications. This superior performance often translates to increased efficiency, reduced weight, enhanced durability, and improved functionality. The deliberate design of these materials distinguishes them from naturally occurring substances or conventional alloys and compounds. The development of advanced materials is a multidisciplinary endeavor, drawing upon expertise in chemistry, physics, nanotechnology, ceramics, metallurgy, and biomaterials, among others.
The applications of advanced materials are vast and span numerous industries. They are integral to advancements in aerospace, healthcare, electronics, energy, and transportation. For example, lightweight and strong composite materials are used in aircraft and automobiles to improve fuel efficiency, while flexible and high-capacity batteries for portable electronics rely on advanced materials. Biomaterials, another category, find applications in medical implants.
A diverse array of materials falls under the umbrella of advanced materials. These include advanced alloys with tailored properties, advanced polymers with unique functionalities, and porous materials with controlled structures. Particulate systems like quantum dots and graphene, novel fibers based on organic or inorganic materials, and composites that combine different materials are also considered advanced. Metamaterials with engineered electromagnetic or acoustic properties and nanomaterials exhibiting responsive, catalytic, optical, or magnetic behaviors further expand this category.
To better understand the landscape of advanced materials, they can be broadly categorized based on their primary function:
Category | Examples | Applications |
Structural Materials | Composites (carbon fiber), Alloys (superalloys), Ceramics, Polymers | Aerospace, automotive, construction, engineering applications requiring strength and stiffness |
Functional Materials | Semiconductors, Superconductors, Piezoelectric materials, Magnetostrictive materials | Electronics, energy harvesting, sensors, actuators |
Biomaterials | Metals, Ceramics, Polymers, Composites (biocompatible and non-toxic) | Medical implants, prosthetics, drug delivery systems |
Advanced Alloys | Intermetallic alloys, Shape memory alloys, High entropy alloys | High-temperature applications, actuators, specialized structural components |
Advanced Polymers | Electroactive polymers, Self-repairing polymers, Copolymers | Flexible electronics, sensors, self-healing applications |
Porous Materials | Microporous, Mesoporous, Macroporous materials | Filtration, catalysis, energy storage |
Particulate Systems | Quantum dots, Supraparticles, Nanoflowers, Graphene | Electronics, photonics, energy storage, biomedical imaging |
Novel Fibers | Organic fibers, Carbon-based fibers, Inorganic fibers | Textiles, composites, reinforcement materials |
Composites | Fiber-reinforced composites, Particle-reinforced composites, Hybrid materials | Lightweight structures, high-strength components across various industries |
Metamaterials | Electromagnetic metamaterials, Acoustic metamaterials | Cloaking devices, improved antennas, acoustic dampening |
Nanomaterials | Responsive nanomaterials, Catalytic nanomaterials, Optical nanomaterials, Magnetic nanomaterials, Nanocarriers | Diverse applications across medicine, electronics, energy, and environmental science, including targeted drug delivery, advanced sensors, and enhanced energy storage |
Export to Sheets
The development and application of advanced materials are crucial drivers of innovation and economic growth, essential for addressing global challenges such as sustainable energy, environmental protection, and healthcare. However, it is also important to consider the full lifecycle of these materials and any potential risks associated with their use. The frequent mention of nanoscale engineering and nanomaterials within the definitions and examples of advanced materials highlights a significant point: engineering at the nanoscale is a key method for achieving the enhanced properties that define advanced materials. Furthermore, the early emphasis on the uncertain hazard profiles and the need for special testing for advanced materials suggests a critical aspect of this field: responsible innovation that considers potential risks alongside the benefits.
Demystifying Nanotechnology: Core Principles and a Glimpse into its Applications
Closely intertwined with the field of advanced materials is nanotechnology, which involves the manipulation of matter with at least one dimension sized from 1 to 100 nanometers (nm). This manipulation at the nanoscale allows for the creation of structures, devices, and systems with novel properties and functions. A nanometer is incredibly small – one-billionth of a meter; to put it in perspective, the head of a pin is about 1 million nanometers wide. At this scale, commonly known as the nanoscale, surface area and quantum mechanical effects become significantly important in determining the properties of matter.
Several core principles underpin the field of nanotechnology. One fundamental aspect is the emergence of size-dependent properties. At the nanoscale, materials can exhibit mechanical, electrical, optical, and chemical characteristics that are distinctly different from those of their bulk counterparts. For example, the increased surface area-to-volume ratio in nanoparticles enhances their chemical reactivity and can lead to unique optical and electronic behaviors due to quantum effects.
Nanotechnology employs various fabrication approaches to create nanoscale structures. These include "bottom-up" methods, where materials and devices are built from molecular components that self-assemble chemically based on principles of molecular recognition. Supramolecular chemistry plays a key role in this approach, designing molecules that automatically arrange themselves into desired configurations due to non-covalent intermolecular forces. Conversely, "top-down" approaches involve constructing nano-objects from larger entities without atomic-level control, often through miniaturization processes. Techniques like nanofabrication and manipulation of nanoparticles are also central to controlling matter at this scale. In fields like nanomedicine, the principles of nanotechnology are applied to control properties such as stability, solubility, bioavailability, and the controlled release of drugs. The theoretical foundations of nanotechnology are complex, drawing from diverse scientific disciplines including intermolecular forces, thermodynamics of small systems, and molecular self-assembly.
The applications of nanotechnology are incredibly broad and continue to expand across numerous sectors. It has been embraced by industries such as information and communications, food technology, energy, and medicine. Nanomaterials offer opportunities for reducing environmental pollution. In medicine, nanotechnology is being used for disease diagnosis, targeted drug delivery, advanced imaging, and tissue engineering. The energy sector benefits from nanotechnology through enhanced solar cells, more efficient energy storage, and improved lighting technologies. Nanotechnology also finds applications in textiles, leading to stain-resistant and more durable fabrics. The sheer breadth of these application areas, spanning from medicine to energy to materials science, underscores that nanotechnology is a truly cross-disciplinary and enabling technology. Furthermore, the distinction between nanoscience, the fundamental study of nanoscale phenomena, and nanotechnology, the practical application of this knowledge, highlights the importance of both basic research and technological innovation in advancing the field.
Powering the Future Sustainably: Enhancing Energy Efficiency
The global focus on sustainable energy has driven significant research into how advanced materials and nanotechnology can enhance energy efficiency across various domains.
Harnessing the Sun's Potential: Advanced Materials and Nanotechnology in Solar Cells
Solar energy, a clean and abundant resource, can be harnessed more effectively through the application of advanced materials and nanotechnology. Traditional solar cells primarily utilize silicon as a semiconductor material. While silicon-based cells offer a good combination of efficiency, cost, and lifespan, researchers are continually exploring alternative materials and nanotechnological approaches to further improve their performance.
One promising alternative is perovskite solar cells. These thin-film cells, named after their characteristic crystal structure, have rapidly advanced in efficiency, now rivaling and even surpassing silicon-based cells in laboratory settings. In 2022 and 2023, scientists developed perovskite-silicon tandem solar cells that achieved efficiencies exceeding 30%. The low cost of raw materials and ease of manufacturing are significant benefits of perovskites. However, their durability remains a challenge, with perovskite cells being fragile and susceptible to degradation from heat, moisture, and oxygen. Nanotechnology is playing a crucial role in addressing this limitation. For instance, surface functionalization techniques using compounds like 5-ammonium valeric acid iodide (5-AVAI) enable the uniform growth of aluminum oxide (Al₂O₃) through atomic layer deposition, creating a robust barrier that significantly improves the stability of perovskite solar cells.
Quantum dots, another area where nanotechnology shines, are nanoscale semiconductors that can absorb and emit light with high efficiency. Their bandgap is customizable, allowing them to collect light from a wider spectrum than traditional materials, thus increasing energy conversion efficiency. Quantum dots can also be paired with other semiconductors like perovskites to optimize the performance of multijunction solar cells, which layer multiple semiconductor materials to capture different wavelengths of light.
Nanotechnology also contributes to solar cell efficiency by enhancing light absorption. Incorporating nanomaterials such as nanostructured silicon or titanium dioxide increases the surface area of solar cells, allowing for more efficient capture of sunlight. Nanocoatings, such as protective layers of nanoparticles, can improve durability by shielding solar cells from environmental factors. Furthermore, even non-specific nanoparticles can boost efficiency by increasing light scattering within the solar cell, effectively trapping more light for conversion. Nanofabrication techniques are essential for creating these intricate nanostructures and thin-film porous templates that further enhance energy conversion. The rapid progress in perovskite solar cell efficiency, especially when combined with silicon in tandem cells, indicates a potential shift in the solar energy landscape. Moreover, nanotechnology provides multiple avenues for improving solar cell performance, from enhancing light absorption at the nanoscale to improving the durability and stability of new materials.
Revolutionizing Energy Storage: The Impact of Nanotechnology on Battery Technology
Efficient energy storage is crucial for the widespread adoption of renewable energy sources and the advancement of electric vehicles. Nanotechnology is revolutionizing battery technology by enabling advanced materials with enhanced performance characteristics.
One key area of focus is increasing energy density, which determines how much energy a battery can store for a given weight or volume. Traditional lithium-ion batteries, while having high energy density compared to other commercial technologies, are being further improved through nanotechnology. Silicon anodes, for example, can store significantly more lithium ions than conventional graphite anodes, leading to higher energy density. However, silicon experiences large volume changes during charging and discharging, which can cause degradation. Nanostructuring silicon into nanowires or nanoparticles helps to mitigate this issue, improving the battery's lifespan. Carbon nanotubes are another advanced material used in battery design. Their strength and lightweight properties help reduce the overall weight of battery packs while increasing conductivity and efficiency.
Nanotechnology also plays a vital role in enhancing power density, which refers to how quickly a battery can deliver energy. Nanoscale electrodes increase the surface area for electrochemical reactions, enabling ultra-fast charging. Advanced electrolyte formulations enhanced with nanoparticles improve ion conductivity, allowing for quicker charge and discharge rates. Furthermore, nanotechnology is facilitating the development of safer and more stable batteries. Solid-state batteries, which replace the flammable liquid electrolyte with a solid material, are a promising next-generation technology often enabled by nanoscale engineering of the solid electrolyte. Lithium-sulfur batteries, with a theoretical energy density significantly higher than lithium-ion, are also being explored, with nanotechnology helping to address challenges related to their stability and lifespan. The consistent emphasis on silicon anodes and carbon nanotubes as key nanomaterials for next-generation batteries highlights their importance. Additionally, the emergence of solid-state and lithium-sulfur batteries, often enabled by nanotechnology, suggests a move towards safer and higher-performance alternatives to traditional lithium-ion batteries.
Smarter Insulation: Advanced Materials and Nanotechnology for Thermal Management
Efficient thermal insulation is essential for reducing energy consumption in buildings and industrial processes. Advanced materials, including those enabled by nanotechnology, offer superior insulation properties.
Aerogels, often referred to as "frozen smoke," are nanoporous materials with exceptionally low thermal conductivity, making them highly effective insulators while being lightweight. Silica nanoparticles are often integrated into the aerogel matrix to enhance its thermal resistance. Aerogel insulation blankets are available for various applications in residential and commercial buildings, providing maximum thermal efficiency with minimal thickness. Vacuum insulation panels (VIPs) also offer high thermal resistance in a thin form factor, meeting the need for space-saving insulation solutions.
Nanotechnology also contributes to smarter insulation through the development of nano-coatings. These coatings can be applied to windows, walls, and roofs to reduce heat transfer, helping to maintain comfortable indoor temperatures and lower energy bills. For example, NanoTech Insulative Coat utilizes NanoTech Insulative Ceramic Particle (ICP)™ technology, which boasts extremely low thermal conductivity and high emissivity, effectively reducing heat transfer in various applications. This type of nanotech insulation is also effective for retrofitting existing structures to modern energy efficiency standards. The ability of nanotechnology to enhance the properties of existing insulation materials and enable new forms like aerogels and nano-coatings is crucial for improving thermal management. Aerogel consistently emerges as a high-performance insulation material, often enabled or enhanced by nanotechnology, indicating its significant potential for energy savings despite potential cost challenges. Furthermore, the trend towards smart and sustainable insulation materials suggests a growing awareness of the environmental impact of building materials and a desire for more dynamic and eco-friendly solutions.
Driving Towards a Greener Tomorrow: Transforming Transportation
The transportation sector is a major contributor to energy consumption and greenhouse gas emissions. Advanced materials and nanotechnology are playing a pivotal role in making vehicles lighter, more efficient, and ultimately more sustainable.
Lightweighting for Efficiency: Advanced Materials in Vehicle Construction
Reducing the weight of vehicles is a direct way to improve fuel efficiency and reduce emissions. Advanced materials with high strength-to-weight ratios are replacing heavier traditional materials like steel and cast iron in vehicle construction.
Aluminum alloys are one such material, offering high strength and corrosion resistance at one-third the weight of steel. They are widely used in engine blocks, chassis, and body panels. Carbon fiber composites are even lighter and stronger than steel, making them ideal for high-performance vehicles and electric vehicles where weight reduction is crucial to compensate for the heavy battery packs. Advanced high-strength steel (AHSS) is engineered for greater strength with less weight compared to traditional steel. Magnesium alloys, being significantly lighter than both steel and aluminum, are used in various components like steering wheels and seat frames. Polymer composites and nanomaterials also offer a combination of lightweight properties, heat resistance, and durability.
Nanotechnology is contributing to lightweighting through the development of nanocomposites. By adding nanoparticles to existing composite materials, their physical and chemical properties, such as strength and corrosion resistance, can be significantly improved while maintaining low density. For electric vehicles, using nanocomposites for the frame can effectively extend their driving range by reducing overall weight. Carbon nanotubes and clay nanocomposites are noted for their strength and lightweight characteristics, making them suitable for vehicle parts and bodies. Carbon fiber composites and aluminum alloys are frequently mentioned as key lightweighting materials, indicating their current and future importance in the automotive industry. Furthermore, nanotechnology is emerging as a significant contributor to vehicle lightweighting by enabling the creation of nanocomposites with superior strength and lower density compared to traditional materials.
Sleek and Efficient: The Role of Advanced Coatings in Transportation
Advanced coatings play a crucial role in improving the efficiency and longevity of vehicles by providing wear resistance, reducing friction, and protecting against corrosion.
Diamond-like carbon (DLC) coatings, often enhanced by nanotechnology, offer exceptional hardness, low friction, and superior wear resistance, making them ideal for engine components and other critical parts in both traditional and racing vehicles. Electroless nickel coatings provide uniform plating on complex components like gears and heat sinks, contributing to their efficiency and reliability. Ceramic coatings, including graphene-based variants, offer excellent chemical resistance, high gloss, and a super slick surface, providing long-lasting protection to the vehicle's exterior. These nanocoatings also often exhibit self-cleaning properties due to their hydrophobic nature, repelling water and dirt.
Nanotechnology is enabling the development of "smart" coatings with functionalities beyond simple protection. Self-healing coatings, for example, can automatically repair minor scratches and chips, maintaining the vehicle's appearance and integrity. Nano-enhanced adhesives are even being developed to replace or outperform welds in certain applications, allowing for stronger bonding between different materials and contributing to lightweighting. The increasing popularity of ceramic and graphene-based coatings for vehicles indicates a growing consumer demand for long-lasting and high-performance surface protection. Moreover, nanotechnology is enabling the development of "smart" coatings with functionalities beyond simple protection, such as self-healing and enhanced adhesion, which can contribute to both efficiency and safety in transportation.
Optimizing Industrial Processes: Efficiency in Manufacturing
Efficiency in manufacturing is paramount for reducing costs, minimizing waste, and promoting sustainability. Advanced materials and nanotechnology are contributing significantly to this goal through innovations in catalysis and tool coatings.
The Catalytic Advantage: Nanotechnology for Enhanced Chemical Reactions
Catalysts are essential for accelerating chemical reactions in numerous industrial processes, from producing fuels and chemicals to controlling emissions. Advanced materials, particularly nanomaterials, are revolutionizing catalyst design, leading to enhanced efficiency, selectivity, and sustainability.
The high surface area-to-volume ratio of nanomaterials provides more active sites for chemical reactions to occur, significantly increasing the reaction rate and efficiency. For example, fuel cell catalysts often involve nanoparticles that enhance the efficiency of clean energy technologies. Nanomaterials like metal nanoparticles, carbon-based nanostructures, and metal oxides are being used in a wide range of catalytic applications, including energy production, environmental remediation, and fine chemical synthesis.
Researchers are also drawing inspiration from nature to design bio-inspired and biomimetic catalysts that mimic the highly selective and environmentally friendly processes found in living organisms. Materials like metal-organic frameworks (MOFs) and zeolites, which resemble natural formations, are used as catalyst supports, enabling complex reactions with minimal environmental impact. Precise control over the size, shape, and composition of nanocatalysts through advanced synthesis techniques is crucial for optimizing their performance. Even the arrangement of nanoparticles on a substrate can significantly impact the selectivity of a catalytic reaction. The consistent emphasis on the high surface area of nanomaterials as a key driver for enhanced catalytic activity highlights a fundamental principle in nanocatalysis. Furthermore, the emergence of bio-inspired catalysts and the use of materials like MOFs indicate a trend towards designing catalysts with enhanced selectivity and environmental sustainability.
Precision and Durability: Advanced Coatings for Tools and Machinery
The performance and lifespan of tools and machinery in manufacturing processes are critical for efficiency. Advanced coatings, including those enhanced by nanotechnology, play a vital role in improving these aspects.
Various types of advanced coatings, such as Titanium Nitride (TiN), Titanium Carbon Nitride (TiCN), Titanium Aluminum Nitride (TiAlN), and Diamond-like Carbon (DLC), are applied to cutting tools to enhance their wear resistance, reduce friction, and improve cutting efficiency. These coatings increase the hardness of the tool surface, allowing for higher cutting speeds and feeds, which ultimately reduces machining time and costs. Nanotechnology is further enhancing the performance of these coatings. For example, carbide tools with diamond nano-coatings maintain their cutting edge for longer periods, resulting in consistent precision and reduced downtime. Multilayer and nanostructured coatings offer improved heat and wear resistance, making them suitable for machining difficult-to-cut materials and composites. Nanocoatings can also provide resistance to chemical corrosion, which is particularly important in certain manufacturing environments, such as machining plastics. The use of nanocoatings on tools can significantly extend their lifespan, reducing the frequency of replacements and contributing to more sustainable manufacturing practices. Diamond-like carbon (DLC) coatings, often mentioned in the context of nanotechnology, stand out for their exceptional hardness and wear resistance, making them ideal for demanding machining applications. Moreover, the development of nanocomposite coatings tailored for specific materials like plastics demonstrates the ongoing innovation in this field to address the unique challenges of different manufacturing processes.
Building a Smarter World: Innovations in Infrastructure
The infrastructure sector, encompassing buildings, bridges, roads, and other essential structures, can greatly benefit from the application of advanced materials and nanotechnology to enhance efficiency, durability, and sustainability.
Self-Healing Structures: Nanotechnology for Resilient Concrete
Concrete, the most widely used construction material globally, is prone to cracking due to various environmental and mechanical stresses. Self-healing concrete, an innovative material enabled by advanced materials and nanotechnology, offers a solution to this problem by automatically repairing cracks, thus extending the lifespan of structures and reducing maintenance costs.
One approach to self-healing concrete involves incorporating dormant bacteria, typically from the Bacillus genus, into the concrete mix. When cracks form and water infiltrates, these bacteria become activated and, through their metabolic processes, precipitate calcium carbonate (CaCO₃), which effectively seals the cracks. This process, known as microbially induced calcium carbonate precipitation (MICCP), can repair cracks up to a certain width, depending on the type of bacteria used. The bacteria are often encapsulated in protective carriers like microcapsules or hydrogels to ensure their survival within the harsh alkaline environment of concrete.
Another method utilizes polymers that are blended with cement to create a composite material capable of self-healing. These polymers form dynamic bonds with the cement, allowing the material to reversibly bond across fissures and heal damage within a short period, often within 24 hours. This approach can also increase the elasticity and strength of the concrete, making it more resistant to cracking.
Nanotechnology plays a significant role in the development of self-healing concrete. Nanomaterials, such as nanoparticles, nanotubes, and nano-coatings, can be incorporated into concrete to enhance its mechanical properties, improve crack recovery, and offer superior resistance to environmental stressors. For example, nano silica can fill the pores in concrete, reducing its permeability and increasing its compressive strength. The prevalence of bacteria-based self-healing concrete research suggests a bio-inspired and potentially more sustainable approach to infrastructure resilience. Furthermore, the use of nanomaterials like nano silica to enhance both the strength and self-healing properties of concrete indicates that nanotechnology can provide multi-functional benefits for infrastructure materials.
Intelligent Building Envelopes: Smart Windows for Energy Conservation
Windows are a significant source of energy loss or gain in buildings. Smart windows, utilizing advanced materials and nanotechnology, offer a dynamic solution to this issue by adjusting their properties, such as transparency or color, in response to external stimuli like light or heat. This ability to control light and thermal transmission enhances comfort and significantly reduces energy consumption for heating and cooling.
Several types of smart window technologies exist, often relying on advanced materials. Electrochromic windows use a small electrical charge to change from transparent to tinted, allowing users to control the amount of light and heat entering a space. Photochromic windows react automatically to sunlight, darkening when exposed to UV rays, similar to transition lenses. Thermochromic windows respond to temperature changes, becoming tinted when exposed to heat, thus reducing solar heat gain. Suspended particle devices (SPDs) use microscopic particles in a fluid that align to allow light through when electricity is applied and scatter light to block it when electricity is removed. Liquid crystal windows also use voltage to control transparency.
Nanotechnology plays a crucial role in enhancing the performance and reducing the cost of smart windows. Vanadium oxide nanocoatings are being developed for energy-efficient thermochromic windows that passively adjust tinting based on window surface temperature. These coatings can either absorb or reflect heat depending on their temperature, effectively self-controlling solar radiation and heat transfer. Nanomaterials like graphene and indium tin oxide (ITO) nanoparticles are being utilized in electrochromic windows to improve coloration efficiencies, switching times, and reduce costs. Researchers are also developing flexible and retrofit smart window films embedded with nanoparticles that can be easily applied to existing windows, making the technology more accessible. The recurring mention of vanadium oxide nanocoatings for thermochromic smart windows indicates its potential as a key material for passive energy-saving window technology. Furthermore, the development of flexible and retrofit smart window films based on nanotechnology suggests a move towards making this energy-saving technology more accessible for existing buildings.
Balancing Progress and Planet: Environmental Implications
The development and use of advanced materials and nanotechnology offer significant potential for environmental benefits, but it is also crucial to consider and address the associated challenges.
The Promise of Green Technologies: Environmental Benefits of Advanced Materials and Nanotechnology
Advanced materials and nanotechnology are contributing to a more sustainable world in numerous ways. By enabling greater energy efficiency in various sectors, these technologies help reduce our reliance on fossil fuels, leading to lower greenhouse gas emissions and a smaller carbon footprint.
The development of sustainable materials, such as nano-cellulose derived from renewable resources, offers alternatives to traditional plastics, helping to mitigate the growing problem of plastic pollution. Nanocomposites, being stronger and lighter, require fewer resources to manufacture and maintain. Nanotechnology also plays a vital role in improving water and air quality through advanced filtration systems and the removal of pollutants. For example, nanomaterials like titanium dioxide can be used to break down toxic gases in the atmosphere. In the energy sector, nanotechnology enhances the efficiency of renewable energy technologies like solar cells and batteries, making them more viable alternatives to fossil fuels. The alignment of nanotechnology applications with the UN Sustainable Development Goals underscores its potential to contribute to global sustainability efforts across various sectors. Moreover, the development of sustainable materials like nano-cellulose as alternatives to traditional plastics demonstrates the potential of nanotechnology to address the pressing issue of plastic pollution and promote a circular economy.
Navigating the Challenges: Addressing Potential Environmental Concerns and Toxicity
While the environmental benefits of advanced materials and nanotechnology are promising, it is essential to acknowledge and address potential challenges and concerns. The unique properties of nanomaterials at the nanoscale can also lead to potential risks for human health and the environment.
Some nanoparticles have shown potential toxicity, with concerns raised about their ability to cross biological membranes and interact with cells and organs in ways that larger particles cannot. Studies have investigated the toxicity of various nanoparticles, including silver, copper oxide, and carbon nanotubes, highlighting potential issues like oxidative stress, inflammation, and genotoxicity. The environmental persistence of some nanomaterials and their potential to accumulate in ecosystems also raise concerns.
Resource scarcity for certain advanced materials, such as lithium used in batteries, is another challenge that needs to be addressed through the development of sustainable alternatives. Ensuring the safe handling and disposal of nanomaterials throughout their lifecycle is crucial to prevent unintended environmental consequences. Thorough risk assessment and the development of nano-specific regulations are necessary to guide the responsible development and use of these technologies. Life Cycle Assessment (LCA) is a valuable methodology for evaluating the environmental impacts associated with all stages of a product's life cycle, from raw material extraction to disposal, and should be applied to advanced materials and nanotechnology to ensure their overall sustainability. The consistent emphasis on the potential toxicity of various nanoparticles highlights the critical need for comprehensive nanotoxicology research and robust regulatory frameworks. Furthermore, the importance of conducting Life Cycle Assessments (LCAs) for advanced materials and nanotechnology underscores the need to evaluate their environmental impact holistically.
Looking Ahead: The Future Landscape of Efficiency
The fields of advanced materials and nanotechnology are dynamic and continue to evolve rapidly, promising even greater enhancements in efficiency across various sectors in the future.
Emerging Trends in Advanced Materials for a Sustainable Future
Several key trends are shaping the future of advanced materials. Additive manufacturing, or 3D printing, is becoming increasingly important for producing complex, near-net-shape components with minimal waste, particularly in energy systems. Metamaterials, engineered with properties not found in nature, are showing promise for improving wireless communications and thermal energy storage. The development of sustainable materials, including bio-based polymers and bamboo, as alternatives to conventional materials is gaining momentum. Smart materials that can respond to environmental changes, such as self-healing concrete and electrochromic windows, are moving from research to practical applications in construction. Lightweighting remains a critical focus, with ongoing research into advanced composites and alloys for transportation and other industries. Material informatics, leveraging artificial intelligence and machine learning, is accelerating the discovery and development of new advanced materials. The convergence of advanced materials research with sustainability goals indicates a strong commitment to developing environmentally friendly and resource-efficient materials. Additionally, the growing interest in smart materials, particularly self-healing concrete and electrochromic windows, suggests a future where infrastructure and buildings can adapt to their environment and automatically repair damage.
Potential Breakthroughs in Nanotechnology to Further Enhance Efficiency
Nanotechnology is poised for numerous breakthroughs that could further enhance efficiency in various fields. In energy, advancements in next-generation batteries with higher energy density and faster charging times, as well as more efficient solar cells based on nanoscale materials like perovskites and quantum dots, are anticipated. Self-healing nanomaterials could revolutionize the durability and longevity of products and infrastructure. Nanofabrication techniques will continue to advance, enabling the creation of materials and devices with unprecedented precision and efficiency in manufacturing. Nanotechnology is also expected to yield significant breakthroughs in environmental remediation, with highly efficient nanomaterials for pollutant removal from air, water, and soil. The development of advanced coatings and materials with unique properties, such as self-cleaning, antimicrobial, and superhydrophobic surfaces, will further enhance efficiency and sustainability across various applications. The strong emphasis on advancements in energy storage and conversion indicates that nanotechnology is expected to play a crucial role in addressing the global energy challenges. Furthermore, the recurring theme of self-healing materials at the nanoscale suggests a future where products and infrastructure can automatically repair damage.
Conclusion: Embracing the Potential of Advanced Materials and Nanotechnology for a More Efficient World
Advanced materials and nanotechnology stand as pillars of innovation in the quest for a more efficient world. Their contributions span a vast array of sectors, from revolutionizing energy generation and storage to transforming transportation, optimizing manufacturing processes, and creating smarter, more resilient infrastructure. By enabling superior performance, reducing waste, and fostering sustainability, these fields are addressing critical global challenges. While potential environmental concerns and toxicity risks associated with these technologies must be carefully navigated through rigorous research and responsible development practices, the promise of advanced materials and nanotechnology to build a more efficient and sustainable future is undeniable. The emerging trends and potential breakthroughs in these dynamic fields offer a glimpse into a world where resources are utilized more effectively, environmental impact is minimized, and technological advancements contribute to a higher quality of life for all.
Sources used in the report
Komentarze